Okmok Middle Scoria
Start: 12000 yBP [1]
Stop: 2050 yBP ± 50 Years [1]
Event Type: Explosive
Description: From Wong (2004): "The Middle Scoria Unit (M.S.) of Okmok Volcano is a basaltic andesite (~ 54 wt. % SiO2) fall deposit that is stratigraphically located between two caldera-forming eruptions (~ 12,000 and 2050 y B.P.). Stratigraphy, geochemistry, vesicularity, and crystallinity data imply that the M.S. erupted in three phases that began with an initial phreatomagmatic phase followed by a sub-Plinian phase caused by rapid ascent of magma. The sequence culminates with short-lived Vulcanian eruptions that demonstrate a strong magmatic influence and a possible decrease in ascent rate. The M.S. covers ~ 300 km2 and averages about 2 m thick. Estimates from this work suggest a total eruptive volume of 1.4 km3, an eruption column ~ 9-14 km high, and a mass discharge rate of ~ 8 x 106 kg/s. These values correspond with the low end value of other sub-Plinian and phreatomagmatic eruptions. The changes seen in the eruptive phases of the M.S. are a result of variations in the magma dynamics and access of external water, likely from snow or ice."
"The M.S. has a uniform composition of 54 wt. % SiO2 (Figure 26 [in original text]). Verifying homogeneous glass analyses was difficult because of the microlite-enriched groundmass. The glass chemistries determined could not be used to systematically constrain relative changes within the eruptive layers (Figure 25 [in original text]). Glass data of the M.S. proved ineffective for identifying heterogeneities or magma mixing on a fine scale. However, the analyzed glass data indicate a relatively homogeneous magma with respect to whole rock compositions because there is no significant change in composition to indicate a re-injection of new magma or magma mixing. However, the eruption of the M.S. can be modeled as a three phase eruption that demonstrates both magmatic and phreatomagmatic eruptive behavior (Figure 28 [in original text])."
"The initial eruptive phase of the Middle Scoria had a phreatomagmatic component, seen in the characteristics of the basal ash. The basal ash is highly fragmented and relatively fine-grained compared to the other layers of the M.S., thus the interaction with water was relatively efficient. Evidence for an initially high, yet short-lived phreatomagmatic component is apparent in the blocky grain morphologies. The water source was likely snow or a glacier that melted and drained away from the vent, exposing the magma to the atmosphere (Wilson and Head, 2002). Although the presence of caldera lakes was likely, the cold temperatures required to inhibit the formation of accretionary lapilli supports snow or glacier conditions. Snow in the winter and a stagnant glacier covering about 1.5 km are present within the current caldera near Cone A (Beget and others, in press). An overlying glacier must be relatively thin (< 150 m) though in order to melt fairly rapidly (Smellie, 2000). When ice melts to water, the initial volume reduction would reduce the overpressure as the eruption progresses. The interaction involves significant transfer of water to steam, which increases condensation, thus lowering the heights achieved by the eruptive clouds because of an increase in density from the volumetric change of water (Wilson and Head, 2002). There is no evidence for near-vent eruptive products, nor was a detailed analysis of componentry undertaken, but identifying sources of external water are still possible. It is possible that the central erupting regime was freely vesiculating in simultaneous production or in rapid succession with a marginal (edge) interaction with water, as was the case for the actively vesiculating nature of the 1790 AD Keanakakoi ash (McPhie, 1990). However, interaction with groundwater would require a significant amount of recharge to produce relatively continuous phreatomagmatism evident in the third eruptive phase. The initially high pressures required for a fast ascent rate would preclude conditions of groundwater entry as the pressure of surrounding groundwater would have to exceed the conduit pressure. This process is also true for magma-aquifer interaction. Interaction with an aquifer would yield high lithic contents, however, this did not happen because dense juveniles are also dominant. The thickness of Jl implies the water source could not have been ubiquitous, thus the caldera floor was likely above the water table."
"The second eruptive phase was likely a continuation of a drying out of the first phase as the rapid ascent and high mass discharge of the magma prevented water interaction. Drying out of the snow or ice would enhance conditions for formation of Plinian eruption plumes (Head and Wilson, 2002). Waning water interaction is apparent by the coarse grain population of J l, which demonstrates purely magmatic fragmentation by vesiculation of a relatively strong eruptive pulse that sustained a high convective column (Walker, 1981). Jl is likely the only true layer that should plot in the sub-Plinian field because it shows no evidence for interaction with water."
"With a decreasing magma ascent and a slightly lower mass discharge, any residual heat would melt the snow/ice and produce a small lake, possibly frozen, for the third phase. The lithic to juvenile-rich layers demonstrate the cyclic nature of intermittent Vulcanian style eruptions. A less gaseous magma with a slightly reduced ascent rate is apparent because of the increase in lithic and dense juvenile clasts, which imply increased water availability. When external water is incorporated into the magma, the vent usually widens (Wilson and Head, 1994). Reverse grading in the lithic rich layers is likely the result of vent widening. A subtle increase in eruptive energy from mass discharge estimates for J2 is evident, and is likely responsible for the reverse grading in juvenile rich layers. The ascent rate varied as newly arriving magma or mixing in the column was ongoing based on increases in vesicularity in the intermittent juvenile rich layers (Houghton and others, 1996), as well as the relatively constant eruptive height estimated for the column that produced LI. Therefore, even though the upper layers are not highly fragmented, they are more consistent with typical Vulcanian style eruptions. The negative correlations found for the crystallinity and vesicularity data demonstrate not only that the ascent of the magma varied, but that the interaction with external water was also variable."
From Beget and others (2005): "At least four significant eruptions of mafic scoria (~54 wt. % SiO2; Wong, 2004) occurred during the time period between the older and younger caldera-forming eruptions and these are mainly found to the northeast and south of Okmok caldera. These units are poorly understood, yet contain evidence for explosive interactions between external water and magma, producing phreatomagmatic deposits. The most extensive of these is described in Wong (2004), and consists of thick deposits of scoria and rock fragments deposited to the south and east of the present caldera."
The Global database on large magnitude explosive volcanic eruptions (LaMEVE; 2017) reports a magnitude of 4.6, bulk eruptive volume of 0.245 cubic km and a dense rock equivalent eruptive volume of 0.145 cubic km for the eruption.
"The M.S. has a uniform composition of 54 wt. % SiO2 (Figure 26 [in original text]). Verifying homogeneous glass analyses was difficult because of the microlite-enriched groundmass. The glass chemistries determined could not be used to systematically constrain relative changes within the eruptive layers (Figure 25 [in original text]). Glass data of the M.S. proved ineffective for identifying heterogeneities or magma mixing on a fine scale. However, the analyzed glass data indicate a relatively homogeneous magma with respect to whole rock compositions because there is no significant change in composition to indicate a re-injection of new magma or magma mixing. However, the eruption of the M.S. can be modeled as a three phase eruption that demonstrates both magmatic and phreatomagmatic eruptive behavior (Figure 28 [in original text])."
"The initial eruptive phase of the Middle Scoria had a phreatomagmatic component, seen in the characteristics of the basal ash. The basal ash is highly fragmented and relatively fine-grained compared to the other layers of the M.S., thus the interaction with water was relatively efficient. Evidence for an initially high, yet short-lived phreatomagmatic component is apparent in the blocky grain morphologies. The water source was likely snow or a glacier that melted and drained away from the vent, exposing the magma to the atmosphere (Wilson and Head, 2002). Although the presence of caldera lakes was likely, the cold temperatures required to inhibit the formation of accretionary lapilli supports snow or glacier conditions. Snow in the winter and a stagnant glacier covering about 1.5 km are present within the current caldera near Cone A (Beget and others, in press). An overlying glacier must be relatively thin (< 150 m) though in order to melt fairly rapidly (Smellie, 2000). When ice melts to water, the initial volume reduction would reduce the overpressure as the eruption progresses. The interaction involves significant transfer of water to steam, which increases condensation, thus lowering the heights achieved by the eruptive clouds because of an increase in density from the volumetric change of water (Wilson and Head, 2002). There is no evidence for near-vent eruptive products, nor was a detailed analysis of componentry undertaken, but identifying sources of external water are still possible. It is possible that the central erupting regime was freely vesiculating in simultaneous production or in rapid succession with a marginal (edge) interaction with water, as was the case for the actively vesiculating nature of the 1790 AD Keanakakoi ash (McPhie, 1990). However, interaction with groundwater would require a significant amount of recharge to produce relatively continuous phreatomagmatism evident in the third eruptive phase. The initially high pressures required for a fast ascent rate would preclude conditions of groundwater entry as the pressure of surrounding groundwater would have to exceed the conduit pressure. This process is also true for magma-aquifer interaction. Interaction with an aquifer would yield high lithic contents, however, this did not happen because dense juveniles are also dominant. The thickness of Jl implies the water source could not have been ubiquitous, thus the caldera floor was likely above the water table."
"The second eruptive phase was likely a continuation of a drying out of the first phase as the rapid ascent and high mass discharge of the magma prevented water interaction. Drying out of the snow or ice would enhance conditions for formation of Plinian eruption plumes (Head and Wilson, 2002). Waning water interaction is apparent by the coarse grain population of J l, which demonstrates purely magmatic fragmentation by vesiculation of a relatively strong eruptive pulse that sustained a high convective column (Walker, 1981). Jl is likely the only true layer that should plot in the sub-Plinian field because it shows no evidence for interaction with water."
"With a decreasing magma ascent and a slightly lower mass discharge, any residual heat would melt the snow/ice and produce a small lake, possibly frozen, for the third phase. The lithic to juvenile-rich layers demonstrate the cyclic nature of intermittent Vulcanian style eruptions. A less gaseous magma with a slightly reduced ascent rate is apparent because of the increase in lithic and dense juvenile clasts, which imply increased water availability. When external water is incorporated into the magma, the vent usually widens (Wilson and Head, 1994). Reverse grading in the lithic rich layers is likely the result of vent widening. A subtle increase in eruptive energy from mass discharge estimates for J2 is evident, and is likely responsible for the reverse grading in juvenile rich layers. The ascent rate varied as newly arriving magma or mixing in the column was ongoing based on increases in vesicularity in the intermittent juvenile rich layers (Houghton and others, 1996), as well as the relatively constant eruptive height estimated for the column that produced LI. Therefore, even though the upper layers are not highly fragmented, they are more consistent with typical Vulcanian style eruptions. The negative correlations found for the crystallinity and vesicularity data demonstrate not only that the ascent of the magma varied, but that the interaction with external water was also variable."
From Beget and others (2005): "At least four significant eruptions of mafic scoria (~54 wt. % SiO2; Wong, 2004) occurred during the time period between the older and younger caldera-forming eruptions and these are mainly found to the northeast and south of Okmok caldera. These units are poorly understood, yet contain evidence for explosive interactions between external water and magma, producing phreatomagmatic deposits. The most extensive of these is described in Wong (2004), and consists of thick deposits of scoria and rock fragments deposited to the south and east of the present caldera."
The Global database on large magnitude explosive volcanic eruptions (LaMEVE; 2017) reports a magnitude of 4.6, bulk eruptive volume of 0.245 cubic km and a dense rock equivalent eruptive volume of 0.145 cubic km for the eruption.
References Cited
[1] Physical volcanology of a sub-plinian and phreatomagmatic eruption at Okmok Volcano, Alaska: implications for explosive mafic volcanism, 2004
Wong, L.J., 2004, Physical volcanology of a sub-plinian and phreatomagmatic eruption at Okmok Volcano, Alaska: implications for explosive mafic volcanism: University of Alaska Fairbanks unpublished M.S. thesis, 117 p.[2] Preliminary volcano-hazard assessment for Okmok Volcano, Umnak Island, Alaska, 2005
Beget, J.E., Larsen, J.F., Neal, C.A., Nye, C.J., and Schaefer, J.R., 2005, Preliminary volcano-hazard assessment for Okmok Volcano, Umnak Island, Alaska: Alaska Division of Geological & Geophysical Surveys Report of Investigation 2004-3, 32 p., 1 sheet, scale 1:150,000.

[3] The Middle Scoria sequence: A Holocene violent strombolian, subplinian, and phreatomagmatic eruption of Okmok volcano, Alaska, 2010
Wong, L.J., and Larsen, J.F., 2010, The Middle Scoria sequence: A Holocene violent strombolian, subplinian, and phreatomagmatic eruption of Okmok volcano, Alaska: Bulletin of Volcanology, v. 72, p. 17-31.Complete Eruption References
Global database on large magnitude explosive volcanic eruptions (LaMEVE), 2012
Crosweller H.S., Arora, B., Brown, S.K., Cottrell, E., Deligne, N.I., Guerrero, N.O., Hobbs, L., Kiyosugi, K., Loughlin, S.C., Lowndes, J., Nayembil, M., 2012, Global database on large magnitude explosive volcanic eruptions (LaMEVE): Journal of Applied Volcanology, v. 1, n. 4, unpaged.
Volcanoes of the World, 2013
Global Volcanism Program, 2013, Volcanoes of the World, v. 4.5.3. Venzke, E (ed.): Smithsonian Institution. Downloaded 2017. http://dx.doi.org/10.5479/si.GVP.VOTW4-2013
Physical volcanology of a sub-plinian and phreatomagmatic eruption at Okmok Volcano, Alaska: implications for explosive mafic volcanism, 2004
Wong, L.J., 2004, Physical volcanology of a sub-plinian and phreatomagmatic eruption at Okmok Volcano, Alaska: implications for explosive mafic volcanism: University of Alaska Fairbanks unpublished M.S. thesis, 117 p.
The Middle Scoria sequence: A Holocene violent strombolian, subplinian, and phreatomagmatic eruption of Okmok volcano, Alaska, 2010
Wong, L.J., and Larsen, J.F., 2010, The Middle Scoria sequence: A Holocene violent strombolian, subplinian, and phreatomagmatic eruption of Okmok volcano, Alaska: Bulletin of Volcanology, v. 72, p. 17-31.

Preliminary volcano-hazard assessment for Okmok Volcano, Umnak Island, Alaska, 2005
Beget, J.E., Larsen, J.F., Neal, C.A., Nye, C.J., and Schaefer, J.R., 2005, Preliminary volcano-hazard assessment for Okmok Volcano, Umnak Island, Alaska: Alaska Division of Geological & Geophysical Surveys Report of Investigation 2004-3, 32 p., 1 sheet, scale 1:150,000.


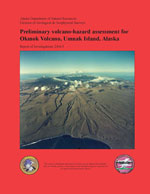